Research Contents
We are exploring various physical and chemical phenomena manifested by the interaction of light and matter from multiple perspectives. By using synchrotron radiation such as HiSOR at Hiroshima University, PF, and UVSOR-III, X-ray free electron laser at SACLA, and the ultrashort pulse laser in the laboratory, we are conducting research on nanofilms, nanoparticles, and bio-related molecules to elucidate the mechanisms of their material functions and biological phenomena at the atomic level, and to synthesize novel nanomaterials and evaluate their physical properties and functions.
The Molecular Photoscience Lab is waiting for you, young novel ideas!
Core-electron excitation dynamics

・Exploiting the locality and element selectivity of core-electron excitation
・Controlling chemical bond breaking reactions by site-selective excitation
・Elucidation of peculiar excited states and relaxation processes by core excitations
One of the most important features of core-electron excitations using soft
X-rays is that it is a localized electronic transition, unlike valence
excitation, which is represented by absorption of visible or UV light.
This is due to the fact that core-electrons (1s orbital electrons) have
a high probability of existence in the innermost orbitals closest to the
atomic nuclei. This feature can be used to selectively excite specific
atoms in a molecule.
This core-excited states retain high energy and inevitably undergo Auger
decays, an electronic relaxation processes. Still unstable, ionic dissociation
reactions occur, resulting in selective chemical bond breaking around the
excited atoms. We have found that such site-selective chemical bond breaking is
significantly observed in self-assembled monolayers (SAMs), in which the
functional groups that serve as reaction sites are regularly arranged on the
topmost surface, and we are investigating the properties of such core-excitation
induced reactions. Furthermore, we are investigating this selective reaction in
detail and applying it to the elucidation of physical properties (especially
conductive properties) at the single molecular level.
Non-contact conductivity evaluation of organic molecules
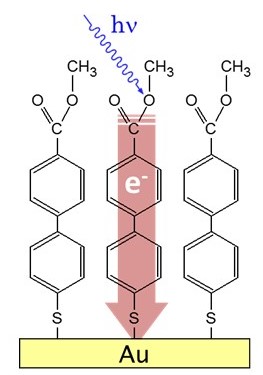
・Developing a technique for non-contact evaluation of electrical conductivity with light
・What happens to Ohm's law at the molecular level?
・Understanding “electric current” in the microscopic world
Electrons flowing in a conducting wire can be easily understood if
treated as particles. As the wire gets narrower and narrower ... How can we
describe the flow of electrons when the conducting wire becomes a conductor of
nanometer (10-9 m) or angstrom (10-10 m; the size of an
atom) size, i.e., a molecular device?
Thus, our goal is to see electrons moving at the molecular level
(the wave functions of fast changing electrons).
After core-electron excitations, the molecules undergo electronic
relaxation processes called Auger decays in a very fast time of femtoseconds
(10-14 - 10-15 s). At this time, if the molecules have
strong electrical connection with a conductor that molecules are in contact
with, the Auger decays are also affected. By spectroscopically observing the
change in electrons emitted by Auger decays, we can study the fast
intramolecular charge transfer with respect to the core-hole lifetime (a few
femtoseconds) (core-hole clock method).
Synthesis of novel metal nanoparticles, junctions, and wires
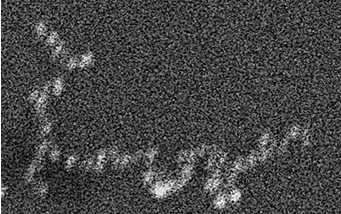
・Synthesis of novel nanoparticles by laser ablation
・Bottom-up nanotechnology using self-assembled monolayers (SAMs)
Nanoparticles, which are materials on the nanometer scale (10-7
- 10-9 m), exhibit properties different from those of materials of
normal size, as exemplified by their catalytic activity.
We have synthesized bare nanoparticles that are stably dispersed in
liquids without being covered with protective molecules, by using a laser
ablation method in which nanoparticles are synthesized by focusing a laser beam
on a metal substrate in a liquid. We have modified these metallic nanoparticles
with functional molecules and synthesized metallic nanoparticle wires by
directly bonding the nanoparticles with molecules. We are also attempting to
synthesize nano-sized alloys and bimetallic nanoparticle wires by mixing
different metals.
By synthesizing such particles, we are conducting research to
develop nanodevice materials and analytical nanoassemblies with controlled
conductivity and particle-particle interactions, and to create novel materials
by functionalizing nanoparticles.
Creation of pseudo-biomembranes and elucidation of protein function and dynamics
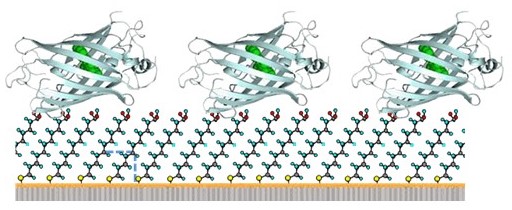
・Creating biotechnology platforms
・Capturing the accurate dynamics of macromolecules
It is long organic molecules (lipids) that form biological tissues
and cells. How is it possible for an assembly of such molecules to maintain
order despite their high degree of freedom? It is molecules such as proteins
that make living organisms function and exchange information. How can they precisely
express their functions in a complex cell? How do they communicate information
(energy)?
Thus, we are interested in accurately observing the motion of
complex or disordered macromolecules. For this purpose, we are developing
observation fields (sample environments) and observation methods (spectroscopic
measurement methods). For example, we are studying the ordered structure of
phospholipid bilayers by utilizing the element selectivity of synchrotron
radiation, and the dense orientation and adsorption of proteins on
self-assembled monolayers (SAMs).
Phase transition dynamics of organic nanocrystals (Solid state polymerization)
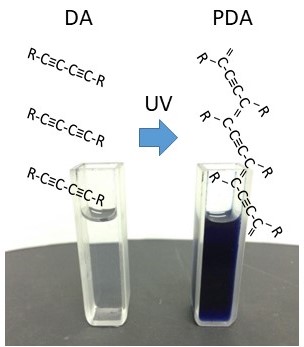
・Capturing the reaction of individual molecules
・Capturing the moment of transition from an individual change to a collective change
Molecules can form crystals by arranging themselves in a regular
pattern, and some of them can cause chemical reactions in their crystalline
state. For example, diacetylene derivatives chemically bond with each other to
form a polymer, polydiacetylene, when irradiated with ultraviolet light, which
is a unique example of an efficient reaction in a solid crystal. By closely
observing such solid-state polymerization reactions in organic nanocrystals, we
hope to capture the changes in individual molecules and the moment of
transition from individual to collective change (phase transition).
To this end, we use a Ti:sapphire laser pulse that shines for very
short period, femtoseconds (10-13 s), to understand the reaction
dynamics on various time scales; electronic state changes on the picosecond
order (10-8 - 10-12 s), and reaction dynamics on the
micro to millisecond order (10-1 - 10-6 s) from dimer to
polymer formation. We expect that such research will lead to the establishment
of new material/material evaluation methods based on the understanding of
solid-state polymerization processes.
Advanced measurement research using X-ray free electron laser (FEL)
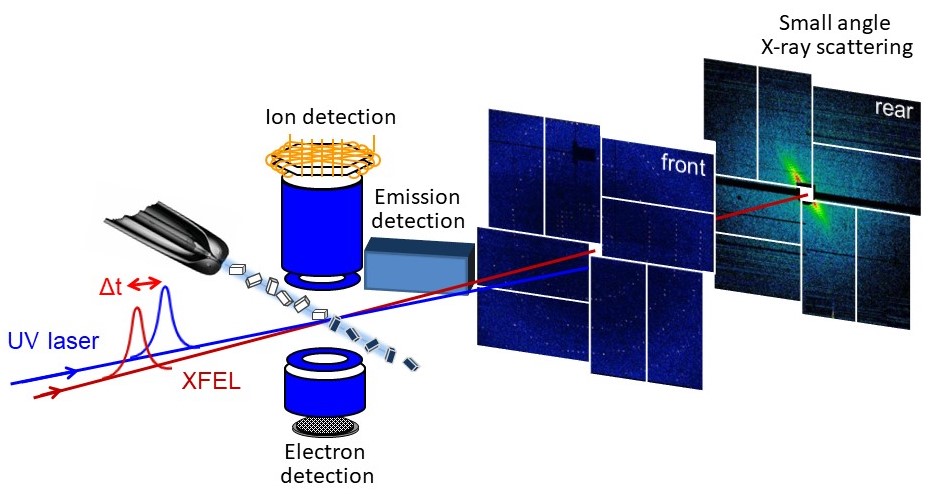
・Uncovering the interaction of powerful X-rays with matter
・Capturing chemical bond changes in real time
Synchrotron radiation and lasers were invented around the same time, 1960s, and have been widely used in scientific research, armed with their respective characteristics. Fifty years later, the dawn of X-ray free electron laser (XFEL), a new type of light that combines the advantages of both, is upon us. This new light source, XFEL, with its short wavelength (X-ray), femtosecond pulse, high brightness, and high coherence, has opened up a new frontier in materials science. By combining such excellent new light and advanced measurement devices, we are challenging collaborative research with domestic and foreign researchers to elucidate new light-matter interactions and real-time measurements of chemical bonding change, targeting atoms, molecules, and their aggregates, clusters.
Light sources
- Synchrotron radiation: HiSOR (BL-6&13), SPring-8, PF, UVSOR, BESSY II (Germany), SOLEIL (France), PETRA III (Germany)
- Optical Laser: Empower, Tsunami & Spitfire (Spectra Physics)
- Free electron laser: SACLA, LCLS(USA)